The Future of Medicine
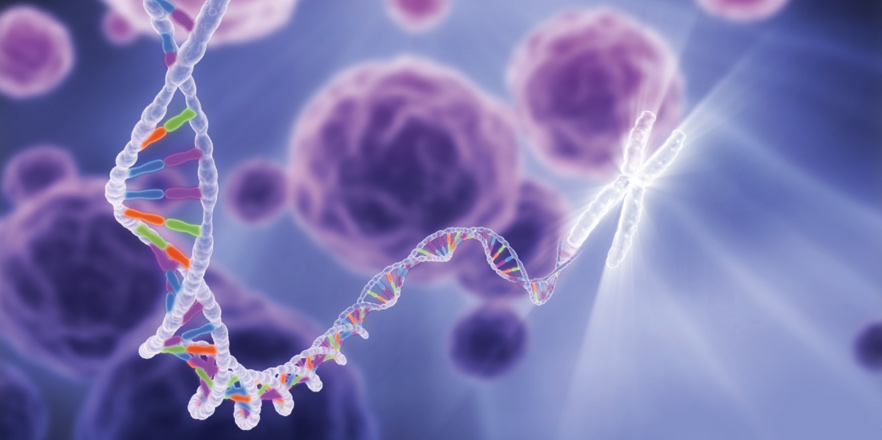
Personalized Medicine. Precision Medicine. Individualized Medicine. Genomic Medicine. Whatever designation you prefer, it is a high-profile topic. Angelina Jolie appeared on the cover of Time magazine to publicize her experience with BRCA-linked breast cancer and, recently disclosed a second elective surgery related to her BRCA status via an op-ed piece in The New York Times. President Obama announced the Precision Medicine Initiative with a proposed 2016 budget of $215 million. Companies are now offering whole genome sequencing and/or tests for selected disease gene panels for as little as a few thousand and a few hundred dollars, respectively. Genomic research has increased dramatically over the last decade and the impact of that research is beginning to appear in clinical practice.
To begin, personalized care has always been a core component of medicine. Physicians assess many “personal” factors including age, gender, physical status and disease characteristics in order to best treat their patients. The new version of Personalized Medicine focuses on the role of genetic variability in key aspects of patient care. The premise: that physicians are better able to predict, diagnose and treat diseases by assessing genetic factors relevant to each patient. While the full potential of Personalized Medicine is yet to be realized, there are already many examples where understanding individual genetic traits has directly improved patient care.
Genetic variability arises through mutations, or variant forms of genes. Each person’s genetic library—the genome—consists of chromosomes, and the chromosomes contain the approximately 21,000 genes comprising that genetic library. The DNA “code” of each gene directs synthesis of proteins responsible for critical cellular functions. When that code is altered by mutational events (faulty DNA duplication during cell division, UV light, carcinogen or radiation exposure), and if DNA repair mechanisms do not correct those alterations, the results are permanent changes, or mutations, of the gene. Mutations are inherited when they occur in parental sperm or egg cells, but also occur in other cells (e.g., mutations due to sun exposure and skin cancers). Those acquired or “somatic” mutations are not passed on to children.
The significance of gene mutations is that they often alter the protein product derived from the mutated gene; in other words, the protein is different from that made via the non-mutated gene. Further, mutations can alter the amount of protein produced (gene expression) in the cell. These in turn can lead to altered functional capabilities of the resultant protein in cells. For certain genes, the mutations and resulting changes in protein function are directly associated with the risk of disease, with the actual cause of disease and/or with individual variation in patient response to medicines used to treat those diseases. This is the basis of Personalized Medicine.
While successes have been documented for many diseases (e.g. cystic fibrosis, Huntington’s Disease), defining genetic causes of cancer has been a particularly exciting area of Personalized Medicine. While cancers arise from a variety of underlying gene mutations, most share a common feature: mutations leading to abnormalities in critical regulatory mechanisms called “cell signaling” pathways. These pathways regulate growth, division and repair of DNA damage in cells. Mutations leading to abnormal function in these pathways can lead to unregulated growth and/or loss of the ability to repair mutations in cellular DNA—hallmarks of cancer. While there are numerous cell-signaling pathways, a number have been well characterized and shown to be the source of many cancer-causing gene mutations. Dozens of such mutations have been reported, including the well-publicized relationship between certain breast cancers and BRCA1/BRCA2 mutations, and the role of mutated HER2 and EGFR in HER2 positive breast cancer and certain lung cancers, respectively.
Completion of the Human Genome Project in 2003 marked a major advance in Personalized Medicine. This decade-long effort, with an investment of approximately $3 billion, was a seminal accomplishment in biomedical research. The immediate product of the HGP was the DNA sequence of a human genome (actually, an amalgam of several individuals). Perhaps the most valuable result of the project has been development of powerful tools and techniques to study more effectively the role of genes in disease and therapy. For example, in 2005, a map of common genetic variants (mutations) across the entire human genome was published. This catalog, called the HapMap, included data from multiple populations and primed research in identifying human disease genes. To date, more than 1,800 disease genes have been discovered utilizing data from the HGP.
The great promise of Personalized Medicine lies in the challenge to develop methods for identification of multiple genes and mutations (as opposed to single gene mutation-disease associations) involved in a given disease across the entire genome, and to do that in large numbers of people. Those challenges are being rapidly met and results from whole genome studies conducted in large numbers of people are starting to appear.
One of the first “large scale” genomics reports comes from investigators who began collecting blood samples from citizens in Iceland in 1998 for whole genome analysis with the goal of studying relationships between genes and diseases in a large, relatively homogenous population. Whole genome sequencing was completed for 2,600 Icelanders, and the data obtained allowed the investigators to infer the DNA sequence for approximately another 100,000 individuals in this unusually homogenous population. A major focus of these studies was to assess the role of multiple gene mutations as well as very rare single gene mutations in major diseases. Early results identified mutations associated with risk of Alzheimer’s disease, atrial fibrillation (irregular heartbeat) and other forms of cardiac disease. More than 10,000 genomes have now been sequenced and those data are currently under study. Additional very large-scale genomic studies are underway and results will be appearing soon.
In addition to dramatic advances in defining the genetic basis of diseases, Personalized Medicine is providing tools for the better selection and use of medicines based on genetic variability among patients (Pharmacogenomics—how genetic factors affect individual responses to drugs). For example, a natural consequence of identifying critical genes and their mutations linked to diseases is that proteins coded by those genes may be targets for drug development. Characterization of disease-involved mutations in the cystic fibrosis (cf) gene led to development of drugs targeted at those mutated cf proteins. Initial clinical trials with patients carrying those mutations show significant improvement in lung function and other indicators of patient status such as weight gain.
Cancer patients are particularly benefiting from an increasing number of drugs targeted at tumors having specific gene mutations in the cell signaling pathways mentioned above. For example, treatment for HER2 positive breast cancer, caused by mutations leading to overproduction of the growth-promoting HER2 protein, has improved markedly, with several drugs and antibodies targeted directly at the HER2 protein. Similar strategies have been applied to many other cancers, including non-small cell lung cancer, leukemias and melanoma, where drugs targeting protein products of genes involved in promoting tumor growth are much more effective in patients with mutations in those genes.
These examples are representative of a major shift in cancer drug development. Rather than studying new agents in specific diseases, long the standard approach, NCI is beginning to evaluate drugs in clinical trials based on the presence of relevant mutations in those growth-promoting pathways, regardless of tumor type (breast, lung, etc.). The expectation with these molecularly targeted drugs is that not only will they be more effective against the relevant cancers, but that side effects will be fewer and less severe than those associated with traditional anticancer agents. The first large clinical trial using this paradigm, the NCI-MATCH trial, focuses on assessing patients for critical mutations in those pathways and directly assigning drugs to patients based on their mutation analysis.
The other major application of Pharmacogenomics to Personalized Medicine is based on the fact that almost all drugs are eliminated from the body by conversion of the parent drug to different forms or “metabolites” in the liver. These metabolic reactions either transform active parent drugs into inactive metabolites or convert inactive parent drugs (prodrugs) into active metabolites. These reactions are catalyzed by protein products of a relatively small number of genes. Many people inherit mutations of these genes and some of the resulting altered proteins are much less active in the liver. As a consequence, active parent drugs metabolized by those proteins will be less effectively converted to inactive forms (with the risk of greater toxicity) or prodrugs will be less effectively converted to active forms (with the risk of underdosing).
There are many examples documenting that patients carrying such mutations of drug metabolizing genes are at greater risk for either increased toxicity or reduced activity of drugs. For example, Plavix is an important anticoagulant used to prevent blood clots in patients who have suffered heart attacks or strokes. Plavix is a prodrug requiring conversion to an active metabolite in the liver. Patients with “reduced function” mutations in the gene coding for the key protein in Plavix activation have significantly less exposure to the active form of the drug (and therefore effectiveness) compared to patients not carrying those mutations. Plavix carries an FDA “Boxed Warning” noting the risks to certain patients carrying those poor metabolizer mutations. FDA suggests alternative treatment approaches for these patients.
Tamoxifen is an important medicine in the treatment of estrogen receptor positive (ER+) breast cancer. The parent drug is relatively inactive, and effective treatment is dependent upon conversion of tamoxifen in the liver to the active metabolite (endoxifen). Patients carrying several “reduced function” variants of the gene coding for the protein responsible for activating the parent drug are at much greater risk for recurrence of their breast cancer because of their inability to produce the active metabolite. Based on those findings, the active metabolite (endoxifen) is now in early clinical trials as a new therapy for ER+ breast cancer, bypassing the genetic limitations for many women.
The impact of Personalized Medicine is clearly evident from the major advances in genomic tools and their application to patient care in the few examples cited here. With these advances there are—as with all new technologies—challenges, cautions and concerns. A significant technical challenge is the sheer volume of data produced by whole genome sequencing and related analyses. The human genome contains approximately 6 billion nucleotide bases to be sequenced in every whole genome sample. Analysis of these enormous data sets is a major task, especially outside of large academic medical centers with substantial Informatics resources. A related issue is how to reduce results from these whole genome assessments to forms readily usable in standard clinical settings.
Another challenge for physicians, medical geneticists and other healthcare professionals is how best to use genetic data in patient settings. There are many gene mutations linked to disease risks for which there is little, if any, “actionable” advice to provide patients. Patients are faced with decisions including what they want to know about their inherited genetic traits, if and when their children should be evaluated for those traits, and decisions about prophylactic procedures that may or may not prevent disease. Relationships of genes, disease causation and treatment options (sometimes none) are often complex. Genetic counseling is often, and should be, a critical component of Personalized Medicine care.
There are many ethical issues related to genetic research and clinical practice. Arguably the most widely discussed is patient data privacy. As genetic information becomes a routine part of (increasingly electronic) patient medical records, the use and sharing of such information (insurance carriers, law enforcement, genetic researchers, etc.) is a major issue with the risk of unauthorized access to those data. Other concerns include genetic discrimination, oversight and regulation of genetic tests, proper informed consent and intellectual property policies.
And finally, as noted earlier, whole genome analyses costing millions of dollars just several years ago are now available for several thousand dollars with genetic tests for specific gene mutations available for several hundred dollars. With this relative affordability, some commercial entities see value in providing these services directly to the consumer, with minimal or no involvement of physicians or other health professionals. Regulating the quality of these services, assessing the quality of the interpretation of the results of such tests, and attempting to insure that consumers know when and how such services are of value is a major challenge for the industry and the government, most notably FDA.
The real impact of Personalized Medicine will be realized when these genetic-based strategies are applied to routine medical practice. In the relatively near future, many of us will provide one blood or saliva sample for whole genome sequencing as a one-time medical test. Our genome sequence (and therefore record of genetic variants for important disease and drug response genes) will be incorporated into our medical history. In the meantime, genetic testing is becoming more common, often in the form of a battery of tests to assess the presence of mutations from multiple genes associated with a single or multiple diseases and/or to assist in the proper selection and use of medicines for those diseases.
At Mayo Clinic, there is now an Individualized Medicine Research Center and Clinic. Within these are many ongoing genomic studies and patient services including whole genome sequencing, multi-gene tests for mutations involved in numerous diseases and in the metabolism of many important medicines, many currently available for patient care. A new initiative involves sequencing 69 genes involved in how patients metabolize or respond to different drugs in 10,000 patients. Results (relevant mutations involved in drug responses) will be incorporated into those patients’ medical records.
Programs similar to these can be found at many academic medical centers. President Obama’s Precision Medicine Initiative proposes, among other goals, to establish a “research cohort” of more than 1 million volunteers to share genetic and other relevant data to accelerate the pace of discovery and translation of genetic variables into practice. The translation of Personalized Medicine research into clinical practice is underway.
Matthew Ames ’70 is emeritus professor and chair of the Department of Molecular Pharmacology and Experimental Therapeutics at the Mayo Clinic College of Medicine.